- Department of Rehabilitation, The Second Xiangya Hospital, Central South University, Changsha, China
Abstract
Osteoarthritis (OA) is a common degenerative joint disease that can lead to chronic pain and disability. The pathogenesis of OA involves chronic low-grade inflammation, characterized by the degradation of chondrocytes, inflammation of the synovium, and systemic low-grade inflammation. This inflammatory response accelerates the progression of OA and contributes to pain and functional impairment. Primary cilia play a crucial role in cellular signal transduction and the maintenance of cartilage matrix homeostasis, and their dysfunction is closely linked to inflammatory responses. Given these roles, primary cilia may significantly contribute to the pathogenesis of OA. This review explores inflammation-associated signaling pathways in OA, including NF-κB, MAPK, JAK/STAT, and PI3K/AKT/mTOR signaling. In addition, we place particular emphasis on cilia-mediated inflammatory modulation in OA. Primary cilia mediate chondrocyte responses to mechanical loading and inflammatory cytokines via pathways including NF-κB, MAPK, TRPV4, and Hedgehog signaling. Notably, alterations in the length and incidence of primary cilia in chondrocytes during OA further underscore their potential role in disease pathogenesis. The identification of biomarkers and therapeutic targets related to primary cilia and inflammatory pathways offers new potential for the treatment and management of OA.
Impact statement
This review is crucial for the field of osteoarthritis research as it unveils the critical role of primary cilia in the inflammatory pathways of osteoarthritis, a previously under explored area. By investigating the complex molecular mechanisms linking primary cilia and inflammation signaling, the review provides new insights into how primary cilia contribute to chronic inflammation and cartilage degradation. These findings may prove valuable for the early diagnosis and targeted treatment of osteoarthritis.
Introduction
Osteoarthritis (OA) is a prevalent chronic joint disease characterized by functional impairment, pain, cartilage degeneration, synovial inflammation, and structural alterations in the subchondral bone, including sclerosis, osteophyte formation, and cystic lesions [1]. As a leading cause of disability and chronic pain globally, OA affects over 500 million people (7% of the world’s population), with a particularly high incidence in the elderly and women [2, 3]. This condition significantly diminishes mobility, physical function, and quality of life in older adults [4]. Despite its widespread impact, the treatment of OA remains challenging, primarily due to the limited understanding of its pathogenesis and the mechanisms underlying its progression [5]. Therefore, there is an urgent need for in-depth research into the key signaling pathways and molecular mechanisms involved in OA, along with the identification of potential biomarkers and therapeutic targets for its various stages.
OA was traditionally regarded as a non-inflammatory joint disease, but recent studies have highlighted the critical role of inflammatory responses in its pathogenesis [6]. Unlike the acute inflammation observed in autoimmune diseases like rheumatoid arthritis (RA), OA is characterized by low-grade, chronic inflammation [4]. Table 1 presents a comparison of osteoarthritis with other types of joint inflammations. A hallmark of the pathological process of OA is synovitis, which involves synovial hyperplasia and the infiltration of inflammatory cells into the synovium. These immune cells release pro-inflammatory factors such as interleukin-1β (IL-1β), tumor necrosis factor-α (TNF-α), and nitric oxide (NO). This inflammatory factors subsequently activate matrix metalloproteinases (MMPs) and aggrecanases, further accelerating cartilage degradation. Ultimately, these processes lead to joint pain and functional impairment [6, 15]. Synovial fluid serves as a multi-functional mediator between the synovium and articular cartilage, and the inflammatory microenvironment of the synovial fluid plays a pivotal role in regulating OA pathogenesis [16]. OA synovial fluid not only drives macrophage polarization toward the pro-inflammatory M1 phenotype [17] but also upregulate catabolic enzymes matrix metalloproteinases (MMPs) [16]. Additionally, mast cells—one of the dominant infiltrating immune cell populations in OA synovium—contribute to synovitis progression and osteophyte formation through the release of pro-inflammatory mediators [18]. In addition to local joint inflammation, systemic inflammation also plays a significant role in the pathogenesis of OA [6]. For instance, obesity, a known risk factor for OA, not only increases the mechanical load on the joints but also triggers systemic low-grade inflammation. This inflammation is mediated by inflammatory factors released by adipose tissue, such as adipokines and other pro-inflammatory cytokines, which further promote the onset of OA [6, 15, 19, 20]. Therefore, inflammation accelerates the progression of OA by fostering chondrocyte degradation, synovial inflammation, and systemic low-grade inflammation, thereby causing pain and functional impairment in patients.
Primary cilia are microtubule-based structures that extend from the surface of cells. They are widely distributed across various cell types, including chondrocytes, synovial cells, and other joint tissue cells, and play an important role in sensing and transmitting mechanical and chemical signals in these cells [21]. Growing evidence indicates that primary cilia are essential for chondrocyte signal transduction and the maintenance of cartilage matrix homeostasis, particularly in the pathogenesis of OA [21]. The direction, length, and number of cilia in chondrocytes are closely linked to OA progression, and their dysfunction may contribute to inflammatory responses and cartilage degeneration [22, 23]. Consequently, regulating primary cilia and their associated signaling pathways not only enhances our understanding of OA pathogenesis but also offers potential therapeutic targets for treatment.
In recent years, there has been increasing attention on the role of primary cilia in the inflammatory signaling processes of OA. This review, therefore, focuses on the involvement of primary cilia in OA-related inflammation. It examines the inflammatory signaling pathways in which primary cilia are involved, as well as the bidirectional regulatory mechanisms between inflammation and primary cilia. The goal of this review is to elucidate the potential roles and mechanisms of primary cilia in OA inflammation. We hope that this article will offer new insights for the treatment of OA.
Inflammatory signaling pathways in osteoarthritis
In OA, sustained chronic inflammation is associated with the degradation of articular cartilage and synovial hyperplasia, promoting joint degeneration and triggering pain responses [24]. These inflammatory reactions are mediated by a series of cytokines, chemokines, and enzymes [25]. Below are several key inflammatory signaling pathways closely associated with OA. The major signaling pathways that regulate inflammation in OA cartilage include NF-κB, JAK/STAT, MAPK, and PI3K/AKT/mTOR, among others [25, 26] (Figure 1).
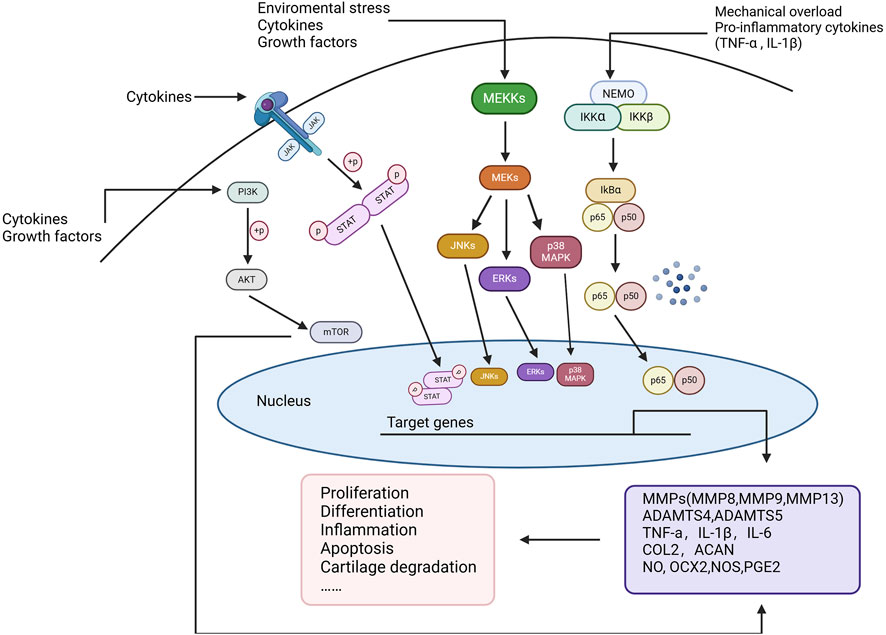
Figure 1. Key inflammatory signaling pathways in OA. Environmental stress, mechanical overload, and pro-inflammatory cytokines (such as TNF-α and IL-1β) activate multiple signaling pathways, including NF-κB, MAPK, JAK/STAT, and PI3K/AKT/mTOR, to regulate cellular inflammatory responses. These pathways, through multi-level phosphorylation cascades, ultimately translocate into the nucleus to regulate the expression of key genes associated with OA progression, such as matrix metalloproteinases (MMPs), ADAMTS enzymes, TNF-α, IL-1β, and IL-6. This process induces cellular proliferation, differentiation, inflammation, apoptosis, and cartilage degradation, playing a pivotal role in OA pathogenesis.
NF-κB signaling pathway
NF-κB is a family of transcription factors that likely plays a critical role in various biological processes, including immune responses, inflammation, cell differentiation, as well as cell proliferation and apoptosis [27]. NF-κB activation occurs mainly through two pathways: the classical and non-classical pathways. In the classical pathway, various immune mediators, such as pro-inflammatory cytokines (e.g., TNF-α and IL-1β), activate the IKK complex (IKKα/β/γ), leading to the phosphorylation and degradation of IκB proteins. This process releases NF-κB dimers (e.g., p65/p50/RelB), which translocate to the nucleus and initiate the transcription of target genes. This process is rapid and reversible [28, 29]. In contrast, the non-classical pathway primarily involves IKKα activation, which leads to the production of p52 and RelB, activating target gene transcription. This pathway is slower and more sustained [30].
In the pathogenesis of OA, NF-κB is a transcription factor extensively involved in the joint inflammation and tissue-destruction processes [5]. Mechanical receptors on the cell membrane of chondrocytes, cytokine receptors, TNFR, and TLRs, activated by pro-inflammatory mediators, mechanical stress, or fibronectin fragments, induce NF-κB signaling [31]. NF-κB signaling promotes the secretion of various degradative enzymes, such as MMPs (including MMP1, MMP9, MMP13) and a disintegrin and metalloproteinases with thrombospondin motifs (ADAMTS, e.g., ADAMTS5), which lead to cartilage degradation [29]. Moreover, NF-κB mediates the production of catabolic cytokines and chemokines, including TNF-α, IL-1β, IL-6, receptor activator of NF-κB ligand (RANKL), and IL-8. This increases the production of MMPs, reduces the synthesis of collagen and proteoglycans, and enhances NF-κB activation through a positive feedback loop [32]. Additionally, NF-κB promotes joint damage by inducing the synthesis of pro-inflammatory molecules such as nitric oxide (NO), cyclooxygenase-2 (COX-2), nitric oxide synthase (NOS), and prostaglandin E2 (PGE2), thereby contributing to cartilage inflammation, the production of catabolic factors, and chondrocyte apoptosis [33, 34]. Furthermore, the NF-κB pathway interacts with other signaling pathways, such as Wnt, to collectively drive the inflammatory response and tissue degeneration in OA [35].
MAPK signaling pathway
Mitogen-activated protein kinases (MAPKs) are a group of serine/threonine protein kinases found in eukaryotes [36]. MAPK signaling is activated by extracellular stimuli such as pro-inflammatory cytokines, growth factors, and oxidative stress. This signaling transduces the extracellular signal into the nucleus via a phosphorylation cascade, regulating various cellular processes [37], such as inflammation responses, catabolism, and degradation of the extracellular matrix, that can have a crucial role in OA development [38]. The extracellular signal-regulated kinase (ERK), c-Jun N-terminal kinase (JNK), and p38 MAPK are the most studied MAPK family members involved in OA pathogenesis. Each cascade consists of at least three enzymes to activate the signaling pathway (MAPKK, MAPK kinase, and MAPK) [39]. Under inflammatory conditions, phosphorylation levels of ERK1, JNK, and p38 MAPK are upregulated. This upregulation stimulates downstream transcription factors, thereby promoting the production of inflammatory cytokines and the progression of inflammatory responses [38]. Inflammatory factors like IL-1 can activate the MAPK signaling pathways, subsequently promoting the expression and activity of MMPs and leading to the degradation of the cartilage [40]. Recent studies have confirmed that both JNK and p38 MAPK signaling pathways are associated with chondrocyte apoptosis in OA [41], while the p38 MAPK and ERK1/2 pathways are closely linked to the cross-talk between bone and cartilage [42, 43].
JAK/STAT signaling pathway
The JAK/STAT signaling pathway consists of three components: Janus kinases (JAKs), signal transducer and activator of transcription proteins (STATs), and tyrosine kinase-associated receptors [44]. This pathway regulates cell proliferation, differentiation, apoptosis, and inflammation [39, 44]. In the pathogenesis of OA, pro-inflammatory cytokines such as IL-1β, IL-6, and TNF-α bind to cell membrane receptors, activating JAK kinases, which in turn phosphorylate STAT transcription factors [45]. The phosphorylated STATs dimerize and translocate to the nucleus, where they bind to promoter regions of target genes to regulate gene expression [44], promoting synovial and cartilage inflammation, as well as the degeneration of cartilage [45]. For example, IL-1β and IL-6 activate JAK/STAT3 signaling in chondrocytes, inducing the expression of MMPs like MMP-13, directly contributing to cartilage matrix degradation and exacerbating cartilage damage [46, 47]. Simultaneously, the JAK/STAT pathway can induce the production of pro-inflammatory factors, such as IL-6, thereby creating a vicious cycle that intensifies synovial inflammation and promotes OA progression [48]. The JAK/STAT signaling pathway also regulates factors such as ELP2 [49] and RANKL [50], which are involved in the differentiation of osteoblasts and osteoclasts. This pathway also induces pathological angiogenesis in subchondral bone through HIF-α/VEGF pathways [51] and factors like EGFL7 [52], thereby exacerbating joint pathological changes.
PI3K/AKT/mTOR signaling pathway
The PI3K/AKT/mTOR signaling pathway consists of PI3K, AKT, and mTOR. When insulin, glucose, and various growth factors and cytokines stimulate the cell membrane, PI3K is activated. PI3K catalyzes the phosphorylation of phosphatidylinositol-4,5-bisphosphate (PIP2) to produce phosphatidylinositol-3,4,5-trisphosphate (PIP3), which activates the downstream AKT protein [53]. The downstream effector of PI3K, mTOR, also functions as a regulator of pro-inflammatory responses in the synovium [53]. In OA pathology, chondrocytes and synovial cells excessively produce inflammatory mediators such as IL-1β and NO [32]. Stimulation by cytokines like IL-1β rapidly phosphorylates PI3K and AKT, leading to the abnormal activation of the PI3K/AKT pathway [54]. This activation increases the production of MMPs by chondrocytes through multiple downstream target proteins [55]. Additionally, protein kinase A (PKA)/AKT can activate NF-κB by affecting the upstream IκB kinase, leading to the phosphorylation and nuclear translocation of NF-κB p65. The interaction between PI3K/AKT and NF-κB enhances the release of inflammatory factor [53].
Primary cilium-mediated inflammatory signaling regulation
Primary cilia participate in a variety of cellular signaling pathways, with the most commonly involved being Hedgehog, Wnt, platelet-derived growth factor (PDGF), transforming growth factor-β (TGF-β), and mechanotransduction [23, 56]. Abnormalities or dysfunction of primary cilia negatively impact cartilage homeostasis, promoting abnormal cartilage remodeling and early-onset OA [57]. Recent studies have shown that primary cilia play a role in the inflammatory processes of OA [23], interacting with several inflammatory signaling pathways, including NF-κB, TRPV4 (Transient Receptor Potential Vanilloid 4), MAPK, and Hedgehog pathways (Figure 2). These inflammatory pathways are involved in the expression of inflammatory factors and the initiation of inflammatory responses, critically affecting the onset and progression of OA. Below, we will describe in detail the relationships between these signaling pathways and primary cilia (Table 2).
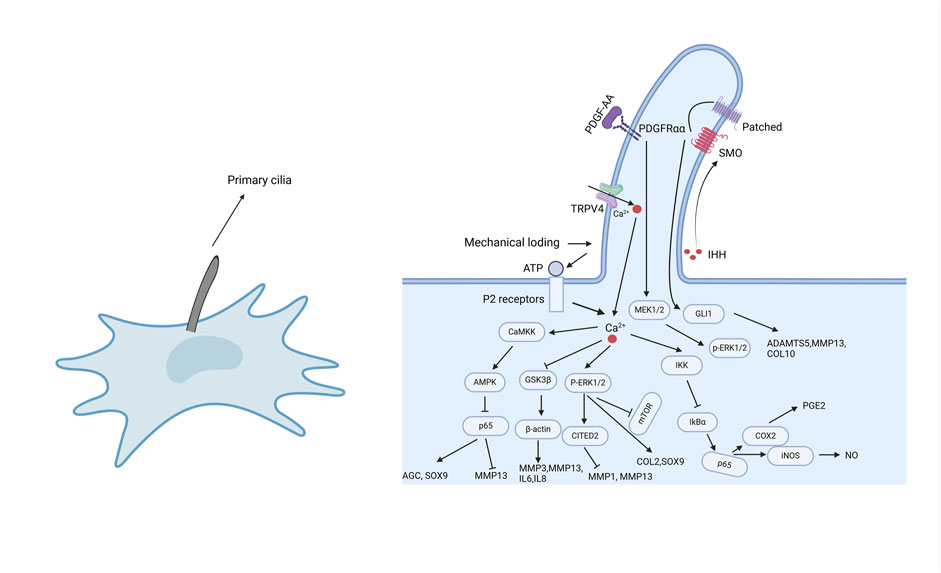
Figure 2. The role of primary cilia in inflammatory responses. As cellular sensory organelles, primary cilia regulate multiple signaling pathways, including NF-κB, MAPK, TRPV4, and Hedgehog pathways. Mechanical loading or external stimuli trigger Ca2+ influx through TRPV4 channels, activating a cascade of signaling events, such as the CaMKK-AMPK-NF-κB axis to regulate inflammation, the GSK3β pathway to influence cytoskeletal dynamics, and the P-ERK1/2 pathway to suppress the overexpression of inflammatory factors. Additionally, primary cilia modulate IHH and PDGFRα signaling, contributing to the regulation of MMPs, ADAMTS enzymes, and inflammation-related factors. These signaling pathways work in concert to regulate cartilage homeostasis, inflammatory responses, and the pathological progression of osteoarthritis.
NF-κB signaling pathway
IL-1β activates a wide range of intracellular inflammatory signaling, largely regulated through either NF-κB signaling, or mitogen activated kinase (MAP) activates or combinations of both. In OA models, damage or dysfunction of primary cilia is often associated with inhibition of the NF-κB pathway [73]. Numerous studies have shown that changes in ciliary proteins, such as IFT88, lead to alterations in the cytoskeletal structure. Since NF-κB relies on an active, dynein-dependent cytoskeletal transport system, it is particularly susceptible to the activation of cytokine-induced pathways [58]. Research by Wann et al. [58, 73]. Revealed how primary cilia influence inflammatory signaling via the NF-κB pathway. The disruption of primary cilia is associated with specific molecular events in the IL-1β-induced NF-κB pathway, leading to the inhibition of COX2 (cyclooxygenase-2) and iNOS (inducible nitric oxide synthase) protein expression [73]. Moreover, although IKK (IκB kinase) activation remains unaffected in cilia-deficient cells, the reduction in IκB phosphorylation delays and decreases IκB degradation, thus affecting the nuclear translocation and binding of NF-κB p65 [58]. Rose BJ et al. [57] also identified the potential key role of heat shock protein 27 (hsp27), which is linked to IKK activity and may be crucial for the regulation of the NF-κB pathway in primary cilia. Meng et al. [59] further demonstrated that YAP signaling regulates NF-κB pathway activation by inhibiting primary cilium expression, thereby blocking IL-1β-induced cartilage matrix degradation and exerting anti-inflammatory effects. However, dysfunction or loss of primary cilia can impair the ability of YAP to regulate the NF-κB pathway.
In conclusion, the disruption of the ciliary protein IFT88 and the loss of primary cilia alter the NF-κB-dependent response to inflammatory signals [58]. Therefore, ciliary proteins in the peripheral cytoplasm may represent a novel component in inflammatory signaling encoding, making them an exciting target for exploring methods to restore physiological inflammation signals in disease processes.
TRPV4 signaling pathway
Mechanical stimuli have anti-inflammatory effects in many tissues [23]. TRPV4, located on the ciliary membrane [74], is one of the primary ion channels that senses mechanical stimuli through primary cilia, detecting mechanical forces in the joint, such as compression, stretching, or fluid shear stress [21]. While TRPV4 channels are widely distributed on the cell membrane of chondrocytes and not limited to primary cilia, the integrity of primary ciliary structure and function appears to play a significant role in TRPV4-mediated calcium responses [75]. Hattori et al. [60] demonstrated that activation of TRPV4 protects joint cartilage through the CaMKK/AMPK/NF-κB signaling pathway. Activation of TRPV4 inhibits the increase in MMP-13 expression induced by IL-1β and the decrease in AGC and SOX9 expression, alleviating cartilage degeneration and inflammation. Similarly, Fu et al. [61] showed that mechanical stimulation activates TRPV4 channels, enhancing HDAC6 expression and ciliary length, thereby mitigating IL-1β-induced NO release, inflammatory responses and cartilage degradation, which positively influences cartilage health. Furthermore, activation of TRPV4 channels in chondrocytes under dynamic compression load promotes anabolic processes and suppresses inflammation [62]. TRPV4 agonists suppress the expression of ADAMTS4 and IL-1 receptor (IL-1R) induced by compressive loading, while TRPV4 antagonists exhibit the opposite effect [63]. These findings suggest that activation of the TRPV4 pathway holds therapeutic potential for inhibiting pro-inflammatory signaling and preventing cartilage degradation in OA.
Other studies, however, have shown that activation of the TRPV4 channel exacerbates the progression of osteoarthritis. Activation of the TRPV4 ion channel increases intracellular calcium levels, which regulate the phosphorylation of GSK3β and inhibit its activity, impairing the ability of chondrocytes to sense the viscoelasticity of the extracellular matrix (ECM) [64]. Furthermore, inactivated GSK3β has been shown to upregulate several transcription factors, including β-catenin, c-Jun, and NF-κB. This upregulation promotes inflammatory responses and cartilage degradation [64, 76]. Sun et al. discovered that M1 synovial macrophage infiltration and TRPV4 expression were significantly increased in OA synovium. Conversely, TRPV4 inhibitors markedly reduced M1 polarization of synovial macrophages, thereby alleviating OA progression, potentially through the ROS/NLRP3 pathway [65]. Additionally, TRPV4 inhibitors downregulate the expression of genes associated with chondrocyte degeneration. In chondrocytes under inflammatory conditions, treatment with TRPV4 inhibitors leads to increased expression of ACAN and COL2, while reducing the expression of MMP13, ADAMTS5, and NOS2 [66].
The role of TRPV4 may be closely related to the timing of its activation, the physiological state of chondrocytes, and the stage of disease progression. Further studies are needed to explore the mechanistic details of TRPV4 and develop precise strategies to regulate its activity in OA treatment to optimize therapeutic outcomes. Overall, these findings highlight the significant role of primary cilia in regulating chondrocyte inflammatory responses via TRPV4 channels.
MAPK signaling pathway
Deciliated chondrocytes exhibit lower basal expression levels of phosphorylated ERK1/2, suggesting that primary cilia may participate in signal transduction through the MAPK/ERK signaling pathway [67]. Studies have shown that under moderate mechanical stimulation, primary cilia, acting as cellular mechanosensors, activate the expression of CITED2 via the ERK1/2 phosphorylation. Such activation downregulates the transcription and expression of matrix-degrading enzymes MMP-1 and MMP-13,thereby exerting an anti-catabolic effect [68]. Additionally, drugs like Icariin [69] and bFGF [77] activate the ERK pathway by enhancing IFT88 expression, inducing the expression of SOX9 and COL2 genes, and promoting cartilage matrix secretion, thereby stimulating chondrocyte proliferation and differentiation while inhibiting inflammatory factors such as MMP3 and MMP9. Xiang et al. [78] showed that after sensing mechanical signals, primary cilia can activate the ERK pathway, further inhibiting the downstream mTOR signaling axis, which not only regulates chondrocyte autophagic activity but also alleviates cartilage degeneration by modulating the expression of inflammatory mediators. The platelet-derived growth factor receptor (PDGFRα), a G-protein-coupled receptor located on primary cilia, activates through binding with PDGF ligands, inducing cellular responses via the downstream MEK/ERK signaling cascade [79].
Hedgehog signaling pathway
The Hedgehog signaling pathway consists of ligands like Sonic Hedgehog (Shh), Indian Hedgehog (Ihh), and Desert Hedgehog (Dhh), and receptors such as Patched (Ptc) and Smoothened (Smo). In the absence of Hedgehog ligands, Ptc inhibits Smo, but upon ligand binding to Ptc, this inhibition is relieved, activating downstream Gli transcription factors and influencing target gene expression [70, 80]. Hedgehog signaling plays a vital role in regulating cell proliferation, differentiation, and tissue morphogenesis [80, 81].
Numerous studies have demonstrated that Hedgehog signaling in chondrocytes requires primary cilia [82], and deletion of IFT88 disrupts primary cilia-mediated Hedgehog signaling [83]. IFT88 maintains the threshold level of Hh signaling under physiological mechanical loading, thereby regulating chondrocyte mineralization [83]. Thompson et al. [84] showed that mechanical loading activates primary cilia-mediated Hedgehog signaling in chondrocytes and promotes ADAMTS-5 expression, emphasizing the close link between primary ciliary length and Hedgehog signaling. This further underscores the importance of primary ciliary structure in Hedgehog signaling transduction and cartilage health. Hedgehog-related genes play key roles in regulating extracellular matrix and inflammatory responses and are involved in the pathogenesis of OA. Yang et al. [85] showed that upregulation of Ihh expression promotes chondrocyte hypertrophy and increases the expression of hypertrophic markers such as collagen X and MMP-13, further promoting cartilage matrix degradation and OA progression. In articular cartilage, IL-1β-induced ciliary elongation may also affect cilia function including mechanotransduction and Hedgehog signaling [86]. In their investigation of the potential of LiCl treatment for OA, Thompson et al. [80] found that LiCl significantly inhibited the Hedgehog signaling pathway, a process directly related to changes in ciliary length. Previous reports have shown that LiCl can suppress IL-1β-induced NF-κB signaling [87]. These studies suggest that LiCl may modulate ciliary length to influence the Hedgehog pathway, thereby playing a role in the regulation of inflammatory signaling.
In summary, the activation and transmission of Hedgehog signaling depend on the structure and function of primary cilia, and changes in primary cilia may be influenced by inflammatory factors like IL-1β. This interaction may play a role in various pathological processes, including the onset and progression of OA.
Cross-regulation of signaling pathways
Primary cilia also engage in cross-regulation with various signaling pathways. According to a review by Pala et al. [88], primary cilia are involved in regulating several signaling pathways, including Hedgehog, Wnt, PDGFR, Notch, and TGF-β. The interactions between these pathways may jointly influence the regulation of inflammation. For example, the interaction between Wnt/β-catenin and NF-κB signaling has been well-documented [89]. Wnt/β-catenin not only reduces the basal expression levels of MMP1, MMP3, and MMP13 but also inhibits NF-κB-induced upregulation of MMPs in human chondrocytes [71, 90]. This indicates that the Wnt/β-catenin pathway can antagonize NF-κB signaling and exert a protective effect. Similarly, activation of TRPV4 can inhibit IL-1β-induced cartilage degeneration and inflammatory responses by activating the CaMKK/AMPK pathway and suppressing NF-κB activation. This process plays a key role in cartilage degradation and the progression of osteoarthritis [60]. Additionally, when chondrocytes are exposed to hypotonic conditions, the expression of TRPV4 channel proteins rapidly increases, triggering a MAPK cascade that leads to ERK1/2 phosphorylation, and in turn, ERK1/2 phosphorylation regulates the endogenous expression of TRPV4 channels. These two processes may interact with each other in a feedback loop [72]. Furthermore, the natural isoflavone glycoside ononin alleviates IL-1β-induced reductions in chondrocyte viability by concurrently downregulating MAPK and NF-κB signaling pathways. Ononin also mitigates the overexpression of inflammatory factors TNF-α and IL-6 and reverses extracellular matrix degradation by inhibiting MMP-13 expression and promoting COL2 expression, thereby improving chondrocyte inflammation [38].
These findings collectively demonstrate the complexity and diversity of the inflammatory response in osteoarthritis. The cross-regulation of multiple signaling pathways involving primary cilia profoundly influences the inflammatory processes associated with osteoarthritis.
Mechanisms of inflammatory damage to primary ciliary function
Primary cilia are involved in modulating inflammatory responses in chondrocytes. When chondrocytes are stimulated by inflammatory cytokines like IL-1β, primary cilia may regulate their function by adjusting ciliary length, a process potentially controlled by IFT protein activity [74]. Primary cilia and IFT (intraflagellar transport) are key in inflammatory signal transduction [86]. IL-1 is one of the most important inflammatory mediators in the formation and progression of OA [23]. Research by Wann et al. [86] showed that exposure to IL-1 for 3 hours increased primary ciliary length by 50% in chondrocytes. This elongation occurs via a protein kinase A (PKA)-dependent mechanism. Furthermore, IL-1-treated chondrocytes exhibited increased release of typical inflammatory mediators such as NO and PGE2. However, in cells with IFT88 mutations leading to ciliary structural loss, the IL-1-induced inflammatory response was significantly diminished, and the progression of OA was correspondingly reduced. These findings suggest that primary cilia are involved in regulating intracellular inflammatory responses.
Histone deacetylase 6 (HDAC6) is a major driver of ciliary disassembly. HDAC6 can induce ciliary disassembly through the deacetylation of α-tubulin and cortactin [91]. Fu [92] and Zhang’s studies [93] indicated that inhibiting HDAC6 promotes microtubule assembly and active elongation, improving the IL-1β-induced inflammatory response. They proposed that the inflammatory environment promotes passive elongation of primary cilia to enhance their mechanotransduction effects, enabling chondrocytes to adapt optimally to inflammation. In contrast, when HDAC6 is inhibited, primary cilia actively elongate to improve mechanotransduction and adjust to their most favorable state.
In summary, primary cilia play a significant role in mediating inflammatory responses through morphological and functional changes. Interestingly, the elongation of primary cilia in response to IL-1 requires the accumulation of hypoxia-inducible factor-2α (HIF-2α) in the cilia [94]. Research by Yang et al. [95] showed that upregulation of HIF-2α promotes OA progression by mediating primary ciliary loss. Another study by Fu et al. [96] also indicated IL-1β-induced elongation of primary cilia in chondrocytes. However, other studies have reported a decrease in the incidence of primary cilia in chondrocytes following IL-1β treatment [75].
Impact of primary cilia on synovitis
Primary cilia serve as sensors to detect changes in the osmotic pressure or chemical composition of synovial fluid and act as signaling transducers to regulate intracellular signaling pathways in chondrocytes [97]. In osteoarthritis, synovial inflammation is a key factor in joint destruction. Fibroblast-like synovial cells (FLS), the main cellular component of the synovium, may promote OA progression by producing pro-inflammatory mediators, such as inflammatory cytokines, nitric oxide (NO), and prostaglandin E2 (PGE2) [98]. FLS respond to the secretion of inflammatory factors by secreting cytokines into the synovial fluid, making them essential components in maintaining the articular cartilage environment [99]. Research by Yuan et al. [100] showed that during inflammatory arthritis, both the length and incidence of cilia in FLS were increased, a change that could enhance the transduction of inflammatory signals to regulate cell function, thereby triggering joint swelling and cartilage damage. Similarly, Estell et al. [99] found that IL-1α significantly increased the average length and incidence of primary cilia in FLS under OA conditions. FLS can sense and respond to mechanical and chemical stimuli in the joint, and changes in their function are associated with OA progression.
Conclusions and perspectives
Inflammation is an integral factor in the pathogenesis of osteoarthritis. Various molecules released by chondrocytes, synovial cells, and immune cells, including cytokines, chemokines, and MMPs, are involved in maintaining cartilage homeostasis [25]. At the same time, the expression of these molecules is regulated by signaling pathways such as NFκB, MAPK, PI3K/AKT, prostaglandins, and nitric oxide [25]. Primary cilia, as sensory organelles, have the ability to detect and regulate physical and chemical signals, making them important mechanoreceptors [74, 101]. There is a close relationship between inflammatory responses, mechanical loading, and cartilage homeostasis [102]. Therefore, targeting the receptors of inflammatory mediators and the mechanotransduction mechanisms of chondrocytes primary cilia may be an effective strategy for directly controlling chondrocyte responses to pathological loads or disease progression [102].
Despite existing research revealing the potential role of primary cilia in inflammatory responses in osteoarthritis, many scientific questions remain unresolved. For example, it is still unclear how primary cilia mediate bidirectional regulation of chondrocyte degradation and regeneration, how primary cilia act as a bridge between different signaling pathways, and how to modulate the function of primary cilia to suppress inflammation and repair cartilage. In conclusion, primary cilia play an important role in the inflammatory response of osteoarthritis and may represent a novel therapeutic target for treating osteoarthritis in the future.
Author contributions
YS designed and wrote the manuscript. ZL, YF, TN, WW and YW revised the manuscript. YS and ZL designed and drew the figures. YK supervised and revised the manuscript. All authors contributed to the article and approved the submitted version.
Funding
The author(s) declare that financial support was received for the research and/or publication of this article. This work was supported by the Natural Science Foundation of China (grant number 82060418).
Conflict of interest
The author(s) declared no potential conflicts of interest with respect to the research, authorship, and/or publication of this article.
Generative AI statement
The authors declare that no Generative AI was used in the creation of this manuscript.
References
1. Martel-Pelletier, J, Barr, AJ, Cicuttini, FM, Conaghan, PG, Cooper, C, Goldring, MB, et al. Osteoarthritis. Nat Rev Dis Primers (2016) 2:16072. doi:10.1038/nrdp.2016.72
2. Hunter, DJ, March, L, and Chew, M. Osteoarthritis in 2020 and beyond: a lancet commission. The Lancet (2020) 396(10264):1711–2. doi:10.1016/S0140-6736(20)32230-3
3. Long, H, Liu, Q, Yin, H, Wang, K, Diao, N, Zhang, Y, et al. Prevalence trends of site-specific osteoarthritis from 1990 to 2019: findings from the global burden of disease study 2019. Arthritis and Rheumatol (2022) 74(7):1172–83. doi:10.1002/art.42089
4. Steinmetz, JD, Culbreth, GT, Haile, LM, Rafferty, Q, Lo, J, Fukutaki, KG, et al. Global, regional, and national burden of osteoarthritis, 1990-2020 and projections to 2050: a systematic analysis for the Global Burden of Disease Study 2021. The Lancet Rheumatol (2023) 5(9):e508–e522. doi:10.1016/S2665-9913(23)00163-7
5. Yao, Q, Wu, X, Tao, C, Gong, W, Chen, M, Qu, M, et al. Osteoarthritis: pathogenic signaling pathways and therapeutic targets. Signal Transduction Targeted Ther (2023) 8(1):56. doi:10.1038/s41392-023-01330-w
6. Robinson, WH, Lepus, CM, Wang, Q, Raghu, H, Mao, R, Lindstrom, TM, et al. Low-grade inflammation as a key mediator of the pathogenesis of osteoarthritis. Nat Rev Rheumatol (2016) 12(10):580–92. doi:10.1038/nrrheum.2016.136
7. Lin, YJ, Anzaghe, M, and Schülke, S. Update on the pathomechanism, diagnosis, and treatment options for rheumatoid arthritis. Cells (2020) 9(4):880. doi:10.3390/cells9040880
8. Scott, DL, Wolfe, F, and Huizinga, TW. Rheumatoid arthritis. The Lancet (2010) 376(9746):1094–108. doi:10.1016/S0140-6736(10)60826-4
9. Porta, S, Otero-Losada, M, Kölliker Frers, RA, Cosentino, V, Kerzberg, E, and Capani, F. Adipokines, cardiovascular risk, and therapeutic management in obesity and psoriatic arthritis. Front Immunol (2021) 11:590749. doi:10.3389/fimmu.2020.590749
10. Talotta, R, Atzeni, F, Sarzi-Puttini, P, and Masala, IF. Psoriatic arthritis: from pathogenesis to pharmacologic management. Pharmacol Res (2019) 148:104394. doi:10.1016/j.phrs.2019.104394
11. Liu, W, Peng, J, Wu, Y, Ye, Z, Zong, Z, Wu, R, et al. Immune and inflammatory mechanisms and therapeutic targets of gout: an update. Int Immunopharmacology (2023) 121:110466. doi:10.1016/j.intimp.2023.110466
12. Cabău, G, Crișan, TO, Klück, V, Popp, RA, and Joosten, LAB. Urate-induced immune programming: consequences for gouty arthritis and hyperuricemia. Immunol Rev (2020) 294(1):92–105. doi:10.1111/imr.12833
13. Wei, Y, Zhang, S, Shao, F, and Sun, Y. Ankylosing spondylitis: from pathogenesis to therapy. Int Immunopharmacology (2025) 145:113709. doi:10.1016/j.intimp.2024.113709
14. Voruganti, A, and Bowness, P. New developments in our understanding of ankylosing spondylitis pathogenesis. Immunology (2020) 161(2):94–102. doi:10.1111/imm.13242
15. Goldring, MB, and Otero, M. Inflammation in osteoarthritis. Curr Opin Rheumatol (2011) 23(5):471–8. doi:10.1097/BOR.0b013e328349c2b1
16. Ingale, D, Kulkarni, P, Electricwala, A, Moghe, A, Kamyab, S, Jagtap, S, et al. Synovium-synovial fluid Axis in osteoarthritis pathology: a key regulator of the cartilage degradation process. Genes (Basel) (2021) 12(7):989. doi:10.3390/genes12070989
17. Kulkarni, P, Srivastava, V, Tootsi, K, Electricwala, A, Kharat, A, Bhonde, R, et al. Synovial fluid in knee osteoarthritis extends proinflammatory niche for macrophage polarization. Cells (2022) 11(24):4115. doi:10.3390/cells11244115
18. Kulkarni, P, Harsulkar, A, Märtson, AG, Suutre, S, Märtson, A, and Koks, S. Mast cells differentiated in synovial fluid and resident in osteophytes exalt the inflammatory pathology of osteoarthritis. Int J Mol Sci (2022) 23(1):541. doi:10.3390/ijms23010541
19. Berenbaum, F, Eymard, F, and Houard, X. Osteoarthritis, inflammation and obesity. Curr Opin Rheumatol (2013) 25(1):114–8. doi:10.1097/BOR.0b013e32835a9414
20. Zhang, Z, Xing, X, Hensley, G, Chang, L, Liao, W, Abu-Amer, Y, et al. Resistin induces expression of proinflammatory cytokines and chemokines in human articular chondrocytes via transcription and messenger RNA stabilization. Arthritis and Rheum (2010) 62(7):1993–2003. doi:10.1002/art.27473
21. Zhang, Y, Tawiah, GK, Wu, X, Zhang, Y, Wang, X, Wei, X, et al. Primary cilium-mediated mechanotransduction in cartilage chondrocytes. Exp Biol Med (Maywood) (2023) 248(15):1279–87. doi:10.1177/15353702231199079
22. Zhou, H, Wu, S, Ling, H, Zhang, C, and Kong, Y. Primary cilia: a cellular regulator of articular cartilage degeneration. Stem Cells Int (2022) 2022:2560441–11. doi:10.1155/2022/2560441
23. Li, X, Guo, S, Su, Y, Lu, J, Hang, D, Cao, S, et al. Role of primary cilia in skeletal disorders. Stem Cells Int (2022) 2022:1–12. doi:10.1155/2022/6063423
24. Scanzello, CR. Role of low-grade inflammation in osteoarthritis. Curr Opin Rheumatol (2017) 29(1):79–85. doi:10.1097/BOR.0000000000000353
25. Chow, YY, and Chin, KY. The role of inflammation in the pathogenesis of osteoarthritis. Mediators Inflamm (2020) 2020:8293921–19. doi:10.1155/2020/8293921
26. Zhou, Q, Ren, Q, Jiao, L, Huang, J, Yi, J, Chen, J, et al. The potential roles of JAK/STAT signaling in the progression of osteoarthritis. Front Endocrinol (Lausanne) (2022) 13:1069057. doi:10.3389/fendo.2022.1069057
27. Hayden, MS, and Ghosh, S. NF-κB, the first quarter-century: remarkable progress and outstanding questions. Genes Dev (2012) 26(3):203–34. doi:10.1101/gad.183434.111
28. Oliver, KM, Garvey, JF, Ng, CT, Veale, DJ, Fearon, U, Cummins, EP, et al. Hypoxia activates NF-κB–Dependent gene expression through the canonical signaling pathway. Antioxid and Redox Signaling (2009) 11(9):2057–64. doi:10.1089/ars.2008.2400
29. Choi, MC, Jo, J, Park, J, Kang, HK, and Park, Y. NF-κB signaling pathways in osteoarthritic cartilage destruction. Cells (2019) 8(7):734. doi:10.3390/cells8070734
30. Sun, SC. The non-canonical NF-κB pathway in immunity and inflammation. Nat Rev Immunol (2017) 17(9):545–58. doi:10.1038/nri.2017.52
31. Yasuda, T. Activation of Akt leading to NF-κB up-regulation in chondrocytes stimulated with fibronectin fragment. Biomed Res (2011) 32(3):209–15. doi:10.2220/biomedres.32.209
32. Kapoor, M, Martel-Pelletier, J, Lajeunesse, D, Pelletier, JP, and Fahmi, H. Role of proinflammatory cytokines in the pathophysiology of osteoarthritis. Nat Rev Rheumatol (2011) 7(1):33–42. doi:10.1038/nrrheum.2010.196
33. Ulivi, V, Giannoni, P, Gentili, C, Cancedda, R, and Descalzi, F. p38/NF-κB-dependent expression of COX-2 during differentiation and inflammatory response of chondrocytes. J Cell Biochem (2008) 104(4):1393–406. doi:10.1002/jcb.21717
34. Marcu, KB, Otero, M, Olivotto, E, Borzi, RM, and Goldring, MB. NF-kappaB signaling: multiple angles to target OA. Curr Drug Targets (2010) 11(5):599–613. doi:10.2174/138945010791011938
35. Ge, XP, Gan, YH, Zhang, CG, Zhou, CY, Ma, KT, Meng, JH, et al. Requirement of the NF-κB pathway for induction of Wnt-5A by interleukin-1β in condylar chondrocytes of the temporomandibular joint: functional crosstalk between the Wnt-5A and NF-κB signaling pathways. Osteoarthritis and Cartilage (2011) 19(1):111–7. doi:10.1016/j.joca.2010.10.016
36. Lan, CN, Cai, WJ, Shi, J, and Yi, ZJ. MAPK inhibitors protect against early-stage osteoarthritis by activating autophagy. Mol Med Rep (2021) 24(6):829. doi:10.3892/mmr.2021.12469
37. Zhou, X, Cao, H, Yuan, Y, and Wu, W. Biochemical signals mediate the crosstalk between cartilage and bone in osteoarthritis. Biomed Res Int (2020) 2020:5720360. doi:10.1155/2020/5720360
38. Xu, F, Zhao, LJ, Liao, T, Li, ZC, Wang, LL, Lin, PY, et al. Ononin ameliorates inflammation and cartilage degradation in rat chondrocytes with IL-1β-induced osteoarthritis by downregulating the MAPK and NF-κB pathways. BMC Complement Med Ther (2022) 22(1):25. doi:10.1186/s12906-022-03504-5
39. Nailwal, NP, and Doshi, GM. Role of intracellular signaling pathways and their inhibitors in the treatment of inflammation. Inflammopharmacology (2021) 29(3):617–40. doi:10.1007/s10787-021-00813-y
40. Mengshol, JA, Vincenti, MP, Coon, CI, Barchowsky, A, and Brinckerhoff, CE. Interleukin-1 induction of collagenase 3 (matrix metalloproteinase 13) gene expression in chondrocytes requires p38, c-jun N-terminal kinase, and nuclear factor κB: differential regulation of collagenase 1 and collagenase 3. Arthritis and Rheum (2000) 43(4):801–11. doi:10.1002/1529-0131(200004)43:4<801::AID-ANR10>3.0.CO;2-4
41. Zhong, M, Carney, DH, Jo, H, Boyan, BD, and Schwartz, Z. Inorganic phosphate induces mammalian growth plate chondrocyte apoptosis in a mitochondrial pathway involving nitric oxide and JNK MAP kinase. Calcif Tissue Int (2011) 88(2):96–108. doi:10.1007/s00223-010-9433-5
42. Prasadam, I, Friis, T, Shi, W, van Gennip, S, Crawford, R, and Xiao, Y. Osteoarthritic cartilage chondrocytes alter subchondral bone osteoblast differentiation via MAPK signalling pathway involving ERK1/2. Bone (2010) 46(1):226–35. doi:10.1016/j.bone.2009.10.014
43. Prasadam, I, van Gennip, S, Friis, T, Shi, W, Crawford, R, and Xiao, Y. ERK-1/2 and p38 in the regulation of hypertrophic changes of normal articular cartilage chondrocytes induced by osteoarthritic subchondral osteoblasts. Arthritis and Rheum (2010) 62(5):1349–60. doi:10.1002/art.27397
44. Leonard, WJ, and O'Shea, JJ. Jaks and STATs: biological implications. Annu Rev Immunol (1998) 16:293–322. doi:10.1146/annurev.immunol.16.1.293
45. Malemud, CJ. Negative regulators of JAK/STAT signaling in rheumatoid arthritis and osteoarthritis. Int J Mol Sci (2017) 18(3):484. doi:10.3390/ijms18030484
46. Lim, H, and Kim, HP. Matrix metalloproteinase-13 expression in IL-1β-treated chondrocytes by activation of the p38 MAPK/c-Fos/AP-1 and JAK/STAT pathways. Arch Pharm Res (2011) 34(1):109–17. doi:10.1007/s12272-011-0113-4
47. Aida, Y, Honda, K, Tanigawa, S, Nakayama, G, Matsumura, H, Suzuki, N, et al. IL-6 and soluble IL-6 receptor stimulate the production of MMPs and their inhibitors via JAK-STAT and ERK-MAPK signalling in human chondrocytes. Cell Biol Int (2012) 36(4):367–76. doi:10.1042/CBI20110150
48. Yang, P, Tan, J, Yuan, Z, Meng, G, Bi, L, and Liu, J. Expression profile of cytokines and chemokines in osteoarthritis patients: proinflammatory roles for CXCL8 and CXCL11 to chondrocytes. Int Immunopharmacology (2016) 40:16–23. doi:10.1016/j.intimp.2016.08.005
49. Xu, CP, Sun, HT, Yang, YJ, Cui, Z, Wang, J, Yu, B, et al. ELP2 negatively regulates osteoblastic differentiation impaired by tumor necrosis factor α in MC3T3-E1 cells through STAT3 activation. J Cell Physiol (2019) 234(10):18075–85. doi:10.1002/jcp.28440
50. Li, CH, Zhao, JX, Sun, L, Yao, Z, Deng, X, Liu, R, et al. AG490 inhibits NFATc1 expression and STAT3 activation during RANKL induced osteoclastogenesis. Biochem Biophysical Res Commun (2013) 435(4):533–9. doi:10.1016/j.bbrc.2013.04.084
51. Giatromanolaki, A, Sivridis, E, Maltezos, E, Athanassou, N, Papazoglou, D, Gatter, KC, et al. Upregulated hypoxia inducible factor-1α and -2α pathway in rheumatoid arthritis and osteoarthritis. Arthritis Res Ther (2003) 5(4):R193–R201. doi:10.1186/ar756
52. Chim, SM, Kuek, V, Chow, ST, Lim, BS, Tickner, J, Zhao, J, et al. EGFL7 is expressed in bone microenvironment and promotes angiogenesis via ERK, STAT3, and integrin signaling cascades. J Cell Physiol (2015) 230(1):82–94. doi:10.1002/jcp.24684
53. Sun, K, Luo, J, Guo, J, Yao, X, Jing, X, and Guo, F. The PI3K/AKT/mTOR signaling pathway in osteoarthritis: a narrative review. Osteoarthritis and Cartilage (2020) 28(4):400–9. doi:10.1016/j.joca.2020.02.027
54. Xie, L, Xie, H, Chen, C, Tao, Z, Zhang, C, and Cai, L. Inhibiting the PI3K/AKT/NF-κB signal pathway with nobiletin for attenuating the development of osteoarthritis: in vitro and in vivo studies. Food Funct (2019) 10(4):2161–75. doi:10.1039/c8fo01786g
55. Chen, J, Crawford, R, and Xiao, Y. Vertical inhibition of the PI3K/Akt/mTOR pathway for the treatment of osteoarthritis. J Cell Biochem (2013) 114(2):245–9. doi:10.1002/jcb.24362
56. Leu, T, Denda, J, Wrobeln, A, and Fandrey, J. Hypoxia-inducible factor-2alpha affects the MEK/ERK signaling pathway via primary cilia in connection with the intraflagellar transport protein 88 homolog. Mol Cell Biol (2023) 43(4):174–83. doi:10.1080/10985549.2023.2198931
57. Rose, BJ, Kooyman, DL, Porter, J, Woolstenhulme, J, Hanson, R, Woodbury, R, et al. NF-κB activation in osteoarthritis occurs independent of primary cilia associated proteins HSP27 and IKK activity. The FASEB J (2020) 34(1):1. doi:10.1096/fasebj.2020.34.s1.06919
58. Mc Fie, M, Koneva, L, Collins, I, Coveney, CR, Clube, AM, Chanalaris, A, et al. Ciliary proteins specify the cell inflammatory response by tuning NFκB signalling, independently of primary cilia. J Cell Sci (2020) 133(13):jcs239871. doi:10.1242/jcs.239871
59. Meng, H, Fu, S, Ferreira, MB, Hou, Y, Pearce, O, Gavara, N, et al. YAP activation inhibits inflammatory signalling and cartilage breakdown associated with reduced primary cilia expression. Osteoarthritis and Cartilage (2023) 31(5):600–12. doi:10.1016/j.joca.2022.11.001
60. Hattori, K, Takahashi, N, Terabe, K, Ohashi, Y, Kishimoto, K, Yokota, Y, et al. Activation of transient receptor potential vanilloid 4 protects articular cartilage against inflammatory responses via CaMKK/AMPK/NF-κB signaling pathway. Sci Rep (2021) 11(1):15508. doi:10.1038/s41598-021-94938-3
61. Fu, S, Meng, H, Inamdar, S, Das, B, Gupta, H, Wang, W, et al. Activation of TRPV4 by mechanical, osmotic or pharmaceutical stimulation is anti-inflammatory blocking IL-1β mediated articular cartilage matrix destruction. Osteoarthritis and Cartilage (2021) 29(1):89–99. doi:10.1016/j.joca.2020.08.002
62. O’Conor, CJ, Leddy, HA, Benefield, HC, Liedtke, WB, and Guilak, F. TRPV4-mediated mechanotransduction regulates the metabolic response of chondrocytes to dynamic loading. Proc Natl Acad Sci U S A. (2014) 111(4):1316–21. doi:10.1073/pnas.1319569111
63. Takeda, Y, Niki, Y, Fukuhara, Y, Fukuda, Y, Udagawa, K, Shimoda, M, et al. Compressive mechanical stress enhances susceptibility to interleukin-1 by increasing interleukin-1 receptor expression in 3D-cultured ATDC5 cells. BMC Musculoskelet Disord (2021) 22(1):238. doi:10.1186/s12891-021-04095-x
64. Agarwal, P, Lee, HP, Smeriglio, P, Grandi, F, Goodman, S, Chaudhuri, O, et al. A dysfunctional TRPV4-GSK3β pathway prevents osteoarthritic chondrocytes from sensing changes in extracellular matrix viscoelasticity. Nat Biomed Eng (2021) 5(12):1472–84. doi:10.1038/s41551-021-00691-3
65. Sun, H, Sun, Z, Xu, X, Lv, Z, Li, J, Wu, R, et al. Blocking TRPV4 ameliorates osteoarthritis by inhibiting M1 macrophage polarization via the ROS/NLRP3 signaling pathway. Antioxidants (Basel) (2022) 11(12):2315. doi:10.3390/antiox11122315
66. Shen, X, Zhang, H, Chen, DT, and Cao, YL. Preliminary study of TRPV4 affects chondrocyte degeneration. Zhongguo Gu Shang (2023) 36(10):990–5. doi:10.12200/j.issn.1003-0034.2023.10.015
67. Subramanian, A, Budhiraja, G, and Sahu, N. Chondrocyte primary cilium is mechanosensitive and responds to low-intensity-ultrasound by altering its length and orientation. The Int J Biochem and Cell Biol (2017) 91(Pt A):60–4. doi:10.1016/j.biocel.2017.08.018
68. He, Z, Leong, DJ, Zhuo, Z, Majeska, R, Cardoso, L, Spray, D, et al. Strain-induced mechanotransduction through primary cilia, extracellular ATP, purinergic calcium signaling, and ERK1/2 transactivates CITED2 and downregulates MMP-1 and MMP-13 gene expression in chondrocytes. Osteoarthritis and Cartilage (2016) 24(5):892–901. doi:10.1016/j.joca.2015.11.015
69. Xiang, W, Zhang, J, Wang, R, Wang, L, Wang, S, Wu, Y, et al. Role of IFT88 in icariin-regulated maintenance of the chondrocyte phenotype. Mol Med Rep (2018) 17(4):4999–5006. doi:10.3892/mmr.2018.8486
70. Thompson, CL, Wiles, A, Poole, CA, and Knight, MM. Lithium chloride modulates chondrocyte primary cilia and inhibits Hedgehog signaling. FASEB J (2016) 30(2):716–26. doi:10.1096/fj.15-274944
71. Ma, B, van Blitterswijk, CA, and Karperien, M. A Wnt/β-catenin negative feedback loop inhibits interleukin-1-induced matrix metalloproteinase expression in human articular chondrocytes. Arthritis and Rheum (2012) 64(8):2589–600. doi:10.1002/art.34425
72. Hdud, IM, Mobasheri, A, and Loughna, PT. Effect of osmotic stress on the expression of TRPV4 and BKCa channels and possible interaction with ERK1/2 and p38 in cultured equine chondrocytes. Am J Physiology-Cell Physiol (2014) 306(11):C1050–C1057. doi:10.1152/ajpcell.00287.2013
73. Wann, AK, Chapple, JP, and Knight, MM. The primary cilium influences interleukin-1β-induced NFκB signalling by regulating IKK activity. Cell Signal (2014) 26(8):1735–42. doi:10.1016/j.cellsig.2014.04.004
74. Tao, F, Jiang, T, Tao, H, Cao, H, and Xiang, W. Primary cilia: versatile regulator in cartilage development. Cell Prolif (2020) 53(3):e12765. doi:10.1111/cpr.12765
75. Wu, S, Zhou, H, Ling, H, Sun, Y, Luo, Z, Ngo, T, et al. LIPUS regulates the progression of knee osteoarthritis in mice through primary cilia-mediated TRPV4 channels. Apoptosis (2024) 29(5-6):785–98. doi:10.1007/s10495-024-01950-9
76. Han, S. Osteoarthritis year in review 2022: biology. Osteoarthritis and Cartilage (2022) 30(12):1575–82. doi:10.1016/j.joca.2022.09.003
77. Zhan, D, Xiang, W, Guo, F, and Ma, Y. Basic fibroblast growth factor increases IFT88 expression in chondrocytes. Mol Med Rep (2017) 16(5):6590–9. doi:10.3892/mmr.2017.7449
78. Xiang, W, Jiang, T, Hao, X, Wang, R, Yao, X, Sun, K, et al. Primary cilia and autophagy interaction is involved in mechanical stress mediated cartilage development via ERK/mTOR axis. Life Sci (2019) 218:308–13. doi:10.1016/j.lfs.2019.01.001
79. Christensen, ST, Pedersen, SF, Satir, P, Veland, IR, and Schneider, L. The primary cilium coordinates signaling pathways in cell cycle control and migration during development and tissue repair. Curr Top Developmental Biol (2008) 85:261–301. doi:10.1016/S0070-2153(08)00810-7
80. Thompson, CL, Patel, R, Kelly, TA, Wann, AKT, Hung, CT, Chapple, JP, et al. Hedgehog signalling does not stimulate cartilage catabolism and is inhibited by Interleukin-1β. Arthritis Res Ther (2015) 17:373. doi:10.1186/s13075-015-0891-z
81. Rux, D, Helbig, K, Han, B, Cortese, C, Koyama, E, Han, L, et al. Primary cilia direct murine articular cartilage tidemark patterning through hedgehog signaling and ambulatory load. Journal of Bone and Mineral Research (2022) 37(6):1097–116. doi:10.1002/jbmr.4506
82. Thompson, CL, Plant, JC, Wann, AK, Bishop, C, Novak, P, Mitchison, H, et al. Chondrocyte expansion is associated with loss of primary cilia and disrupted hedgehog signalling. Eur Cells Mater (2017) 34:128–41. doi:10.22203/eCM.v034a09
83. Coveney, CR, Zhu, L, Miotla-Zarebska, J, Stott, B, Parisi, I, Batchelor, V, et al. Role of ciliary protein intraflagellar transport protein 88 in the regulation of cartilage thickness and osteoarthritis development in mice. Arthritis Rheumatol (2022) 74(1):49–59. doi:10.1002/art.41894
84. Thompson, CL, Chapple, JP, and Knight, MM. Primary cilia disassembly down-regulates mechanosensitive hedgehog signalling: a feedback mechanism controlling ADAMTS-5 expression in chondrocytes. Osteoarthritis and Cartilage (2014) 22(3):490–8. doi:10.1016/j.joca.2013.12.016
85. Yang, Z, and Song, L. Correlation between Indian hedgehog and damage degree of knee osteoarthritis cartilage. Zhonghua Yi Xue Za Zhi (2014) 94(31):2434–7. doi:10.3760/cma.j.issn.0376-2491.2014.31.010
86. Wann, AK, and Knight, MM. Primary cilia elongation in response to interleukin-1 mediates the inflammatory response. Cell Mol Life Sci (2012) 69(17):2967–77. doi:10.1007/s00018-012-0980-y
87. Minashima, T, Zhang, Y, Lee, Y, and Kirsch, T. Lithium protects against cartilage degradation in osteoarthritis. Arthritis and Rheumatol (2014) 66(5):1228–36. doi:10.1002/art.38373
88. Pala, R, Alomari, N, and Nauli, SM. Primary cilium-dependent signaling mechanisms. Int J Mol Sci (2017) 18(11):2272. doi:10.3390/ijms18112272
89. Du, Q, and Geller, DA. Cross-regulation between Wnt and NF-κB signaling pathways. Forum Immunopathological Dis Ther (2010) 1:155–81. doi:10.1615/forumimmundisther.v1.i3.10
90. Feng, J, Zhang, Q, Pu, F, Zhu, Z, Lu, K, Lu, WW, et al. Signalling interaction between β-catenin and other signalling molecules during osteoarthritis development. Cell Prolif (2024) 57(6):e13600. doi:10.1111/cpr.13600
91. Ran, J, Yang, Y, Li, D, Liu, M, and Zhou, J. Deacetylation of α-tubulin and cortactin is required for HDAC6 to trigger ciliary disassembly. Sci Rep (2015) 5:12917. doi:10.1038/srep12917
92. Fu, S, Thompson, CL, Ali, A, Wang, W, Chapple, J, Mitchison, H, et al. Mechanical loading inhibits cartilage inflammatory signalling via an HDAC6 and IFT-dependent mechanism regulating primary cilia elongation. Osteoarthritis and Cartilage (2019) 27(7):1064–74. doi:10.1016/j.joca.2019.03.003
93. Zhang, Y, Tawiah, GK, Zhang, Y, Wang, X, Wei, X, Chen, W, et al. HDAC6 inhibition regulates substrate stiffness-mediated inflammation signaling in chondrocytes. Acta Biochim Biophys Sinica (2023) 55(12):1987–98. doi:10.3724/abbs.2023144
94. Wann, AK, Thompson, CL, Chapple, JP, and Knight, MM. Interleukin-1β sequesters hypoxia inducible factor 2α to the primary cilium. Cilia (2013) 2(1):17. doi:10.1186/2046-2530-2-17
95. Yang, Q, Zhou, Y, Cai, P, Fu, W, Wang, J, Wei, Q, et al. Up-regulated HIF-2α contributes to the Osteoarthritis development through mediating the primary cilia loss. Int Immunopharmacology (2019) 75:105762. doi:10.1016/j.intimp.2019.105762
96. Fu, S, Meng, H, Freer, F, Kwon, J, Shelton, JC, and Knight, MM. Sub-toxic levels of Co2+ are anti-inflammatory and protect cartilage from degradation caused by IL-1β. Clin Biomech (2020) 79:104924. doi:10.1016/j.clinbiomech.2019.12.006
97. Relucenti, M, Miglietta, S, Covelli, E, Familiari, P, Battaglione, E, Familiari, G, et al. Ciliated cell observation by SEM on the surface of human incudo-malleolar-joint articular cartilage: are they a new chondrocyte phenotype? Acta Oto-Laryngologica (2019) 139(5):439–43. doi:10.1080/00016489.2019.1575520
98. Han, D, Fang, Y, Tan, X, Jiang, H, Gong, X, Wang, X, et al. The emerging role of fibroblast-like synoviocytes-mediated synovitis in osteoarthritis: an update. J Cell Mol Med (2020) 24(17):9518–32. doi:10.1111/jcmm.15669
99. Estell, EG, Murphy, LA, Silverstein, AM, Tan, AR, Shah, RP, Ateshian, GA, et al. Fibroblast-like synoviocyte mechanosensitivity to fluid shear is modulated by interleukin-1α. J Biomech (2017) 60:91–9. doi:10.1016/j.jbiomech.2017.06.011
100. Yuan, G, Yang, ST, and Yang, S. Regulator of G protein signaling 12 drives inflammatory arthritis by activating synovial fibroblasts through MYCBP2/KIF2A signaling. Mol Ther - Nucleic Acids (2023) 31:197–210. doi:10.1016/j.omtn.2022.12.017
101. Muhammad, H, Rais, Y, Miosge, N, and Ornan, EM. The primary cilium as a dual sensor of mechanochemical signals in chondrocytes. Cell Mol Life Sci (2012) 69(13):2101–7. doi:10.1007/s00018-011-0911-3
Keywords: osteoarthritis, primary cilia, inflammatory responses, chondrocytes, inflammatory signaling pathways
Citation: Sun Y, Luo Z, Fu Y, Ngo T, Wang W, Wang Y and Kong Y (2025) Primary cilia and inflammatory response: unveiling new mechanisms in osteoarthritis progression. Exp. Biol. Med. 250:10490. doi: 10.3389/ebm.2025.10490
Received: 04 January 2025; Accepted: 11 April 2025;
Published: 28 April 2025.
Copyright © 2025 Sun, Luo, Fu, Ngo, Wang, Wang and Kong. This is an open-access article distributed under the terms of the Creative Commons Attribution License (CC BY). The use, distribution or reproduction in other forums is permitted, provided the original author(s) and the copyright owner(s) are credited and that the original publication in this journal is cited, in accordance with accepted academic practice. No use, distribution or reproduction is permitted which does not comply with these terms.
*Correspondence: Ying Kong, a29uZ3lpbmcxNTAyQGNzdS5lZHUuY24=